Peering behind the quantum mechanical curtain
Researchers from the Institute for Theoretical Physics used a more general formulation of quantum mechanics to study a breakdown of adiabaticity.
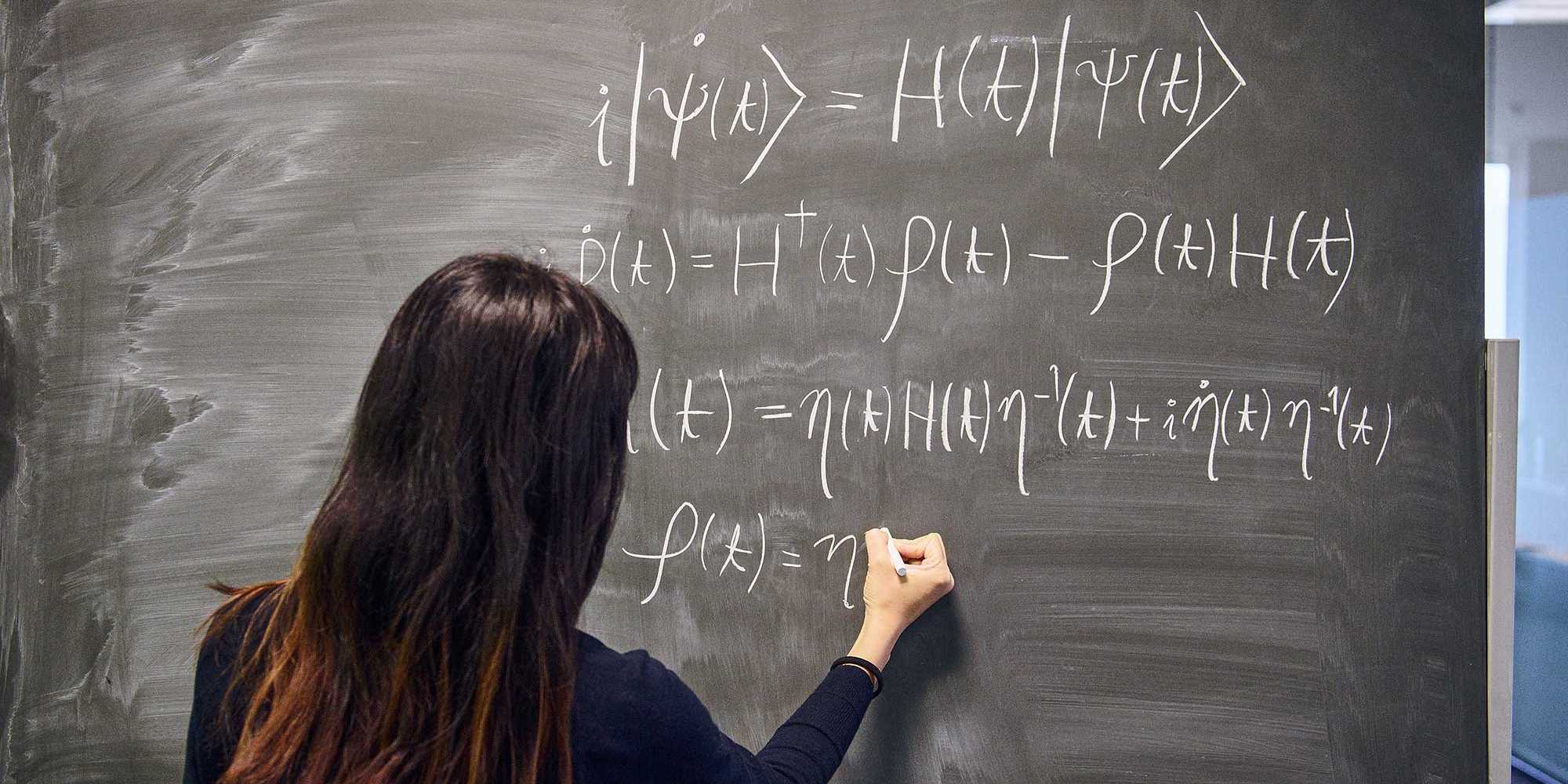
The theory of quantum mechanics (QM) is far from finished business: since the early days of its development, it has prompted questions and discussions around its mathematical foundations and interpretations. In standard QM, observables – that is, physical properties of a quantum system that can be measured – are described by Hermitian operators, which have real spectra as well as complete and orthogonal sets of eigenvectors. Working with Hermitian operators and Hamiltonians captures extremely well crucial aspects of the theory such as the effect of measurement. Nevertheless, the requirement of Hermitian Hamiltonians is not set in stone. In fact, researchers have been studying what happens when it's relaxed and non-Hermiticity can bring about non-unitary time evolution and complex eigenvalues.
Non-Hermitian systems offer a stimulating playground for new physics, as already shown by the unconventional phenomena observed in the laboratory. Away from the bench, theorists work to establish consistent mathematical formulations of non-Hermitian quantum mechanics. Karin Sim, her doctoral advisor Dr Ramasubramanian Chitra, and their colleagues from ETH Zurich and Stockholm University have now put to the test a framework for non-Hermitian QM that's based on a specific time-dependent operator referred to as a quantum metric. Their findings, recently published in Physical Review Letters, shed new light on foundational aspects of quantum mechanical theory and the consequences of non-Hermiticity.
A quantum metric
Sim and colleagues consider a non-stationary Hilbert space endowed with a time-dependent, positive-definite operator ρ that makes it possible to map this Hilbert space, where a system's temporal evolution is generated by a non-Hermitian Hamiltonian, to another Hilbert space where the Hamiltonian is Hermitian and non-Hermiticity is encoded in ρ. It turns out that the role of the metric operator ρ in the study of non-Hermitian systems is far from simple, as the researchers show in the paper.
After introducing the metric and spelling out how to compute the expectation value of a system's observable, Sim and collaborators look at a collection of two-level non-Hermitian systems parametrised by momentum k and driven in time. The interest of this type of system is that it's exactly solvable, meaning that its temporal evolution can be studied analytically between its two Hermitian asymptotic limits. Within the metric framework, the goal is to study the temporal evolution of the system when parity-time (PT) symmetry – which describes the combination of spatial reflection and time reversal operations – is preserved or spontaneously broken. Indeed, for this kind of parametrised system there exist singularities in the parameter space where PT symmetry breaks. At these singularities, known as exceptional points, two or more eigenvalues and their corresponding eigenvectors coalesce.
As a first testbed for the metric framework, Sim and colleagues look at the evolution of the normalised state of the system and find notable discrepancies between the predictions obtained with and without the metric for certain parameter regimes. In the metric framework, the expectations values of observables are weighed by ρ and constitute physically meaningful time-dependent expectation values. In an alternative and well-known approach, which the researchers call the normalisation method, the expectation values of operators are computed through simple normalisation by the time-dependent norm of the corresponding quantum state.
With respect to state evolution, agreement between the metric and normalisation methods is only found when non-Hermiticity is very weak; as soon as non-Hermiticity becomes relevant, the state evolves differently in the two considered frameworks even when PT symmetry is preserved. Sim and collaborators conclude that this is because the metric plays an integral role in the evolution of the quantum state. When the researchers calculate the expectation value of the spin operator, they also find different values depending on the chosen framework. Only with the metric does the expectation value of the spin have a definite parity with respect to momentum k; this finding is consistent with an additional characterisation of the symmetry of the Hamiltonian in the two frameworks, where the researchers report an analogous discrepancy.
A frozen behaviour
Sim and colleagues investigate next what happens when the applied drive becomes increasingly slow, which in quantum mechanical terms corresponds to the adiabatic limit. They define defects as spin flips of the two-level systems that take them away from their initial states. In the metric framework, they find that the density of these defects is characterised by a non-zero contribution that comes exclusively from those two-level systems that manifest spontaneous PT symmetry breaking. This means that defects arise no matter how slowly the system is driven through an exceptional point.
The finding represents a violation of quantum adiabaticity, a phenomenon previously reported in experimental studies: in the standard Hermitian case, the density of defects tends to zero in the adiabatic limit. The researchers stress that this breakdown of adiabaticity, which they call defect freezing, is only recovered in the metric framework – working with the normalisation method fails to capture this unusual behaviour.
According to Sim and collaborators, the defect freezing effect (or its absence) is a consequence of the parity of the spin expectation value, which in turn means that the metric is the crucial ingredient that enables them to capture this feature of the system when studying it within their metric framework. The violation of quantum adiabaticity may not seem so surprising if one thinks that non-Hermitian Hamiltonians describe systems that are intrinsically out of equilibrium. Still, these findings suggest that more work remains to be done – in and out of the laboratory – on understanding the role of the metric operator in formulations of non-Hermitian quantum mechanics.
Reference
Sim, K., Defenu, N., Molignini, P. & Chitra, R. Quantum Metric Unveils Defect Freezing in Non-Hermitian Systems. Phys. Rev. Lett. 131, 156501 (2023). external page DOI:10.1103/PhysRevLett.131.156501