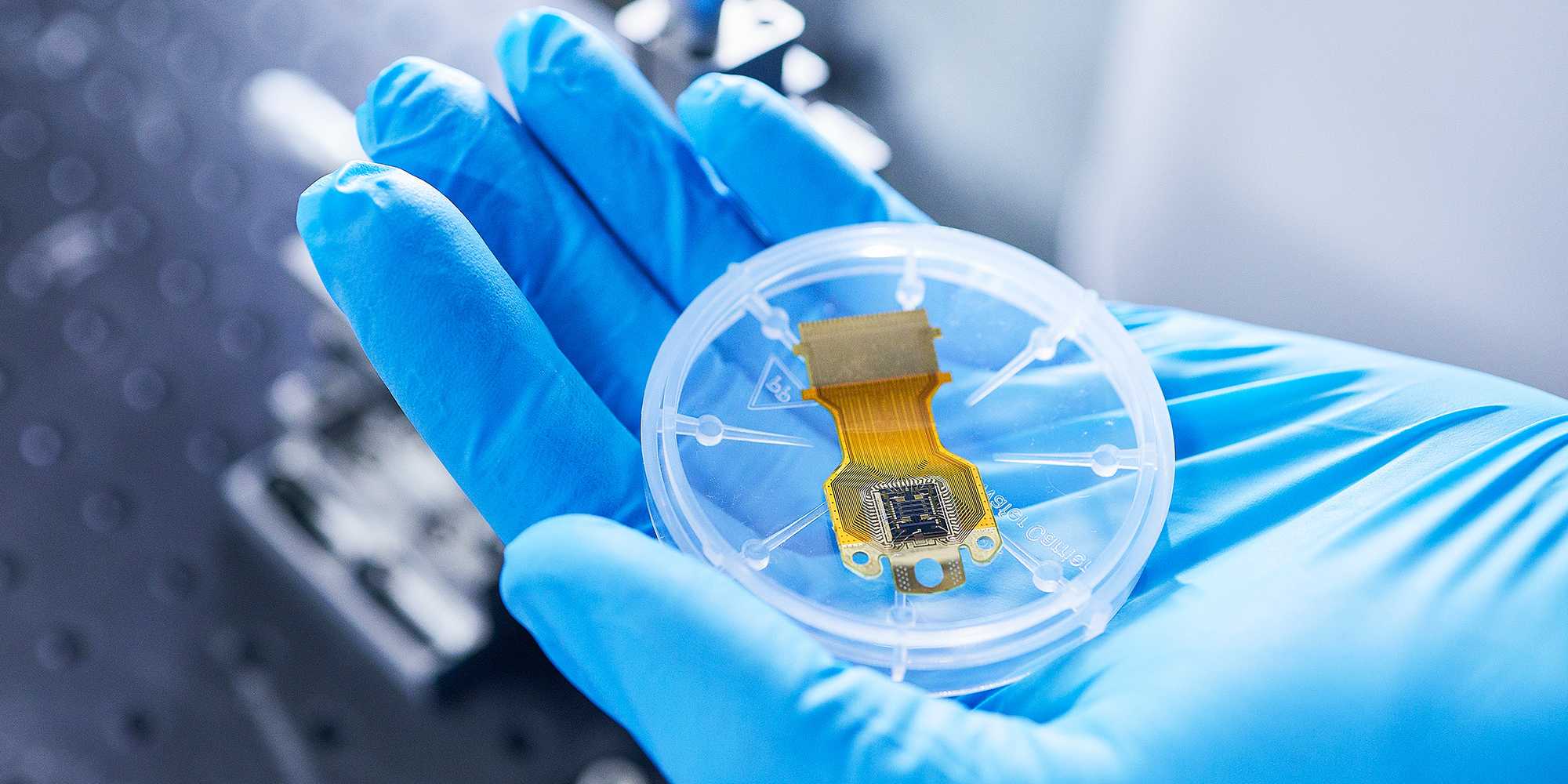
Vacuum fields break into materials engineering
Researchers have shown how electronic correlations in two-dimensional materials can be manipulated through electromagnetic vacuum field fluctuations in a cavity, opening new possibilities for materials research with cavity quantum electrodynamics.
Physics counts many deceitfully simple scenarios. Consider the electromagnetic vacuum, where no photons are present, and you'll find that its emptiness is only apparent: there exist vacuum field fluctuations that are challenging to probe directly but manifest themselves in a variety of phenomena, from spontaneous emission to the Casimir effect. Or, consider electrons moving in two dimensions in the presence of an electrical current and an applied magnetic field perpendicular to the plane of the electrons: lower the temperature of the system and you'll see that the resistivity associated with the resulting electron current takes on quantised values corresponding to integer or fractional multiples of the so-called von Klitzing constant – a phenomenon known as the quantum Hall effect.
The quantum regime of the Hall effect and vacuum field fluctuations are the protagonists of a study authored by Dr Josefine Enkner, Lorenzo Graziotto and collaborators from ETH Zurich, the Université Paris Cité and the École normale supérieure in France, and Princeton and the Flatiron Institute in the US. The findings presented in this work, which has just been published in Nature journal, illustrate how vacuum fields in a cavity can be harnessed to manipulate strongly correlated electron phases in two-dimensional (2D) materials.
The setup used by Enkner, Graziotto and colleagues features a gallium arsenide 2D electron gas in a quantum well heterostructure – identified as the Hall bar – on top of which hovers a mobile cavity in the form of a complementary split-ring resonator carved out of a gold metallic layer. The vacuum fields in the cavity interact with the electron gas: their intensity can be tuned by adjusting the distance between the resonator and the Hall bar. By applying a strong perpendicular magnetic field, the team studied strongly correlated fractional quantum Hall phases through resistance measurements, performed longitudinally along the bar and across it, as the distance between cavity and electron gas is varied. The findings reveal that the cavity enhances some Hall phases, meaning that the characteristic plateaux observed in plots of the Hall resistance – the one measured across the bar – as a function of the magnetic field widen for fractional states 5/3, 7/5 and 4/3.
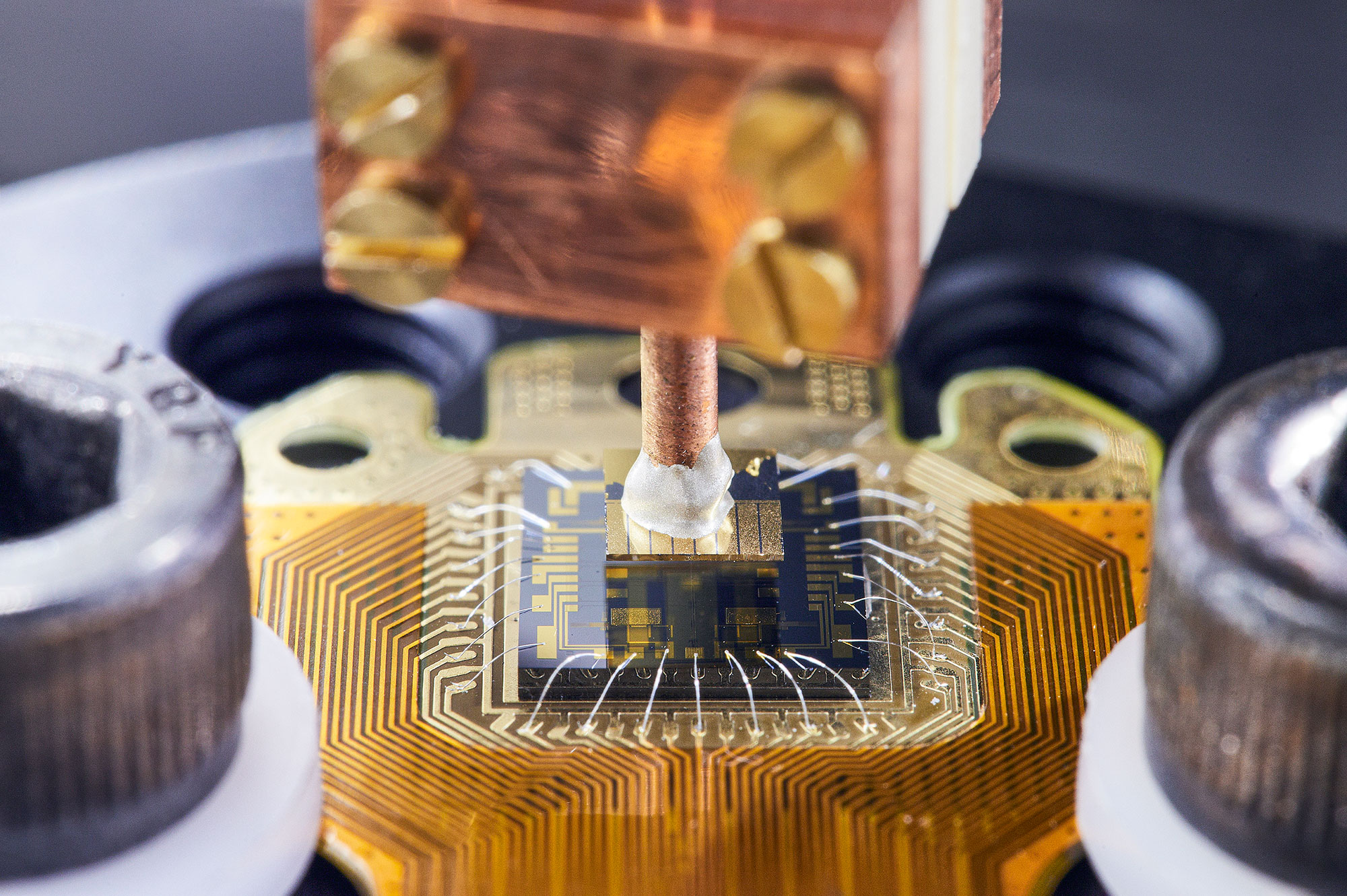
This demonstration made use of a modified design for the setup, which previously saw the cavity fabricated onto the Hall bar. In the present experiment, the Hall bar is encircled by four pillars, etched onto the heterostructure, which prevent physical contact between the bar and the cavity's gold plane while acting as alignment references for the cryogenically cooled system. The new design is notable in that the resonator is thus self-referenced: cavity and electron gas are entirely decoupled from one another. This feature was crucial to study the fractional Hall phases and exclude that the observed behaviour comes from something other than the vacuum field fluctuations. According to Enkner and Graziotto, the self-referencing feature will be even more important for potential future experiments with more complex 2D materials.
To find a theoretical grounding for their observations, Enkner, Graziotto and their colleagues from the Faist Scalari group teamed up with long-term collaborator Cristiano Ciuti and his PhD student Dalin Boriçi. Indeed, the paper presents a simplified theoretical model based on long-range interactions mediated by virtual cavity photons to capture what happens to the fractional Hall phases. At the base of this model is the fact that fractional Hall states derive from electron-electron interactions, hence the cavity must be mediating additional interactions through an induced long-range attractive electron-electron potential.
The team is already busy investigating other fractional Hall phases with their setup. In the longer term, this line of research could lead to a "cavity materials engineering" approach where certain material properties – from crucial topological features to superconductivity – are modified thanks to cavity-confined electromagnetic vacuum fields.
Reference
Enkner, J. et al. Tunable vacuum-field control of fractional and integer quantum Hall phases. Nature (2025). external page DOI:10.1038/s41586-025-08894-3