Rediscovering quantum mechanics through teaching
No two lecturers are likely to teach quantum mechanics in the same way, but many will agree that its richness and intrinsic peculiarities make for a wonderful playground for teaching experimentation.
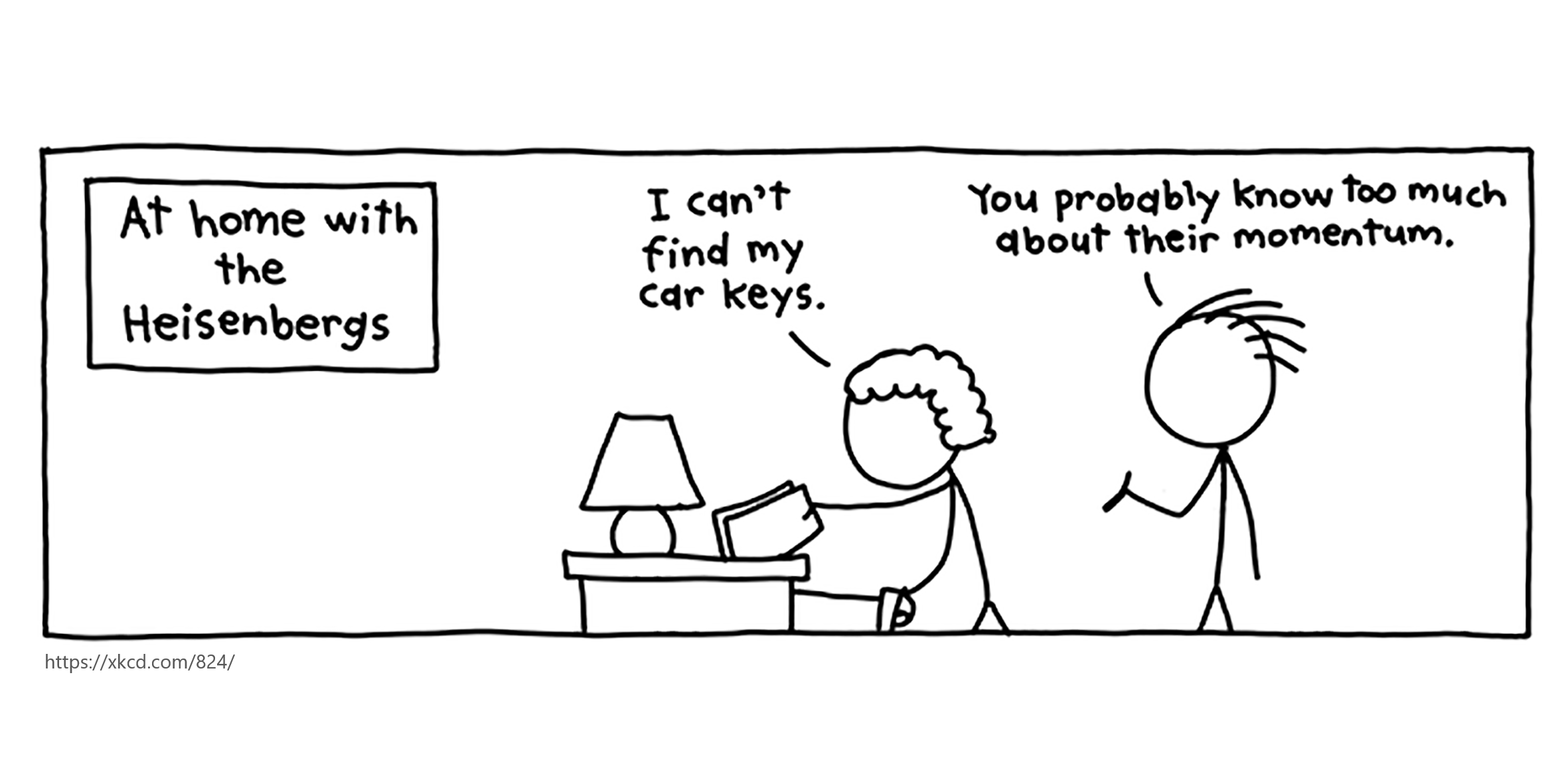
"In quantum mechanics it doesn't matter how knowledgeable you are – every time you look into a topic you find yourself thinking about it differently," says theoretical particle physicist Charalampos Anastasiou, who currently teaches quantum field theory and recently taught quantum mechanics. "It's nice to see students get surprised over and over again," he adds.
Quantum mechanics at ETH Zurich
The Department of Physics offers three main courses on quantum mechanics (QM) at BSc level: Physics III, Quantum Mechanics I and Quantum Mechanics II. The latter course is aimed at students in Theoretical Physics, whereas the first two must be taken by all physics BSc students; all three courses can also be taken by students from other departments. Lecturers change over the years, so that each of them has the chance to reshape the course according to their style and views on the subject.
Physics III is a broad course that covers optics, statistical mechanics and quantum mechanics. When experimental quantum physicist Yiwen Chu first taught it, she knew she wanted to find a thread connecting these seemingly disparate topics. Eventually, she settled on the key ideas of measurement and uncertainty. "Uncertainty shows up differently in the three topics that make up the course: we talk about resolution in imaging systems, we consider probabilistic processes in statistical mechanics, and then in quantum mechanics we see how uncertainty is intrinsically related to the physical properties of an object through measurement," she explains. Physics III provides the first dip into QM and, for example, illustrates its importance for atomic physics; Quantum Mechanics I and II allow students to delve deeper into the theory and to become comfortable with the formalism of QM, which gives them powerful tools to study single-particle and, later on, many-particle systems of bosons and fermions (see below for additional details on each course).
A theory unlike others
As much as QM may be viewed as a well-established theory that can be learned through lectures and an ample choice of textbooks, it has fundamental features that set it apart from other physical theories. "In special relativity you have a good starting point, you know, a set of axioms such as the speed of light in vacuum that's constant and the relativity principle, from which you can derive the whole theory. In quantum mechanics the situation is very different," says theoretical quantum physicist Renato Renner, who has just taught Quantum Mechanics I. "At some point you introduce a Hilbert space and say that the state of a quantum system is a vector in this space. One could ask: why do we use Hilbert spaces, why do we represent states as vectors? All of this comes out of the blue," he adds.
Such legitimate questions may be why some QM courses and textbooks adopt a mixture between a historical and a phenomenological approach, discussing experiments that challenge classical intuition and presenting QM as the new framework that makes sense of these puzzling observations. But this polished view of quantum mechanical theory doesn't quite reflect the way it was developed a hundred years ago. At the time scientists like Max Born, Werner Heisenberg and Wolfgang Pauli carried out much exploratory work and tried out many strategies, as one realizes when browsing their rich correspondence. "Today we read post-selected work," observes Renner, and this post-selection makes it all look even more arbitrary. If one is to rule out the historical or phenomenological introduction to QM, one can look at the theory with hindsight: within the quantum mechanical mathematical framework, the outcomes and predictions one can formulate about quantum systems appear to match experimental observations. In retrospect, it's thus perfectly acceptable to speak of Hilbert spaces and quantum states. "Neither approach is satisfactory to me," says Renner, who openly questions the view according to which QM is a supremely well-tested theory. "As soon as you consider thought experiments that are beyond our technical abilities in the lab – what happens if you put a larger object into a superposition state, say – you realise that there isn't an agreement on how to apply the theory to certain scenarios, and this is connected to its incomplete axiomatic structure."
Trying something different
The challenge of presenting QM through a coherent story despite its unsteady foundations can prompt lecturers to explore different angles and course structures, so that teaching the subject becomes an experiment of its own. Chu decided to introduce the Dirac notation when she first taught Physics III. "I know this wasn't done before my time, but I think that only working with wavefunctions deemphasizes some important aspects of quantum mechanics," she says.
Renner became preoccupied with how the QM courses should prepare students for later courses on quantum information (QI) and quantum field theory (QFT). "The way one teaches quantum mechanics is usually, and by design, somewhat incompatible with quantum information. In standard quantum mechanics, one says that the state of a physical system is a property of the system itself, whereas in quantum information the state is the knowledge one has about the system, which brings it closer to a probability distribution. This incompatibility is rarely addressed," he explains. He decided to redesign the Quantum Mechanics I course and found it to be more challenging than he initially thought. "Some quantum field theory concepts cannot be reconciled with the quantum information standpoint. In quantum field theory, for example, you talk about a measurement as something that happens in an asymptotic future, in a collider experiment, so you don't care about how the measurement influences the field. By contrast, measurement in quantum information is an essential concept. It's difficult to find a common denominator," he says.
To narrow the divide between the basics of QM, QI and QFT, Renner opted for an operational introduction to quantum mechanical theory that requires advanced mathematical tools and concepts such as Lie algebra. He's conscious that he may be asking a lot from his students. "At the beginning of the course they told me, what you tell us has nothing to do with what we saw in Physics III," he says. A similar approach was adopted by Philipp Kammerlander, who heads the Quantum Center and teaches a quantum physics course for students with limited physics knowledge. This course is part of the ETH Master in Quantum Engineering: the course's structure and lecture notes were originally developed by physicist Lídia del Rio. "The issue with many standard introductions to quantum mechanics is that they don't enable students to solve Schrödinger's equation or work out what the expected measurement statistics for a given experiment are," says Kammerlander. This led him to an operational starting point similar to Renner's. "I tell students, this is how we model quantum systems mathematically, this is how we describe a state, this is what observables are and how we measure them, find their statistics and study their evolution." Kammerlander is aware that this head-on strategy comes at a cost. "Wanting to be formal and mathematically rigorous from the beginning, I know I cannot do justice to everything I discuss. I introduce concepts where I say, just take it for now and work with it, then we'll make more sense of it. This happens again and again, so students have to be patient in my course."
Looking ahead
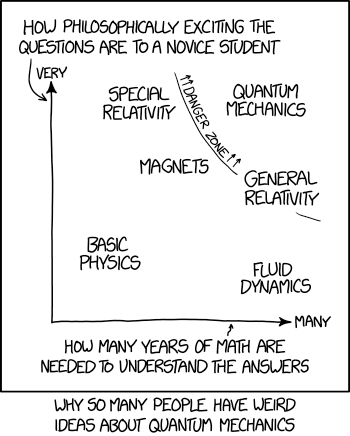
Kammerlander's approach to teaching QM must take into account his students' profiles. "My course isn't meant for those who will go on to become particle physicists or string theorists. My students will take the quantum information processing course and maybe even the quantum information theory course, and as soon as you start to delve into quantum algorithms and so on, nobody will take the time to explain the formalism of the theory," he says. Students completing the Master in Quantum Engineering may well join the ranks of the 'quantum workforce', landing jobs in quantum technology (QT) startups or in larger companies with dedicated QT research units.
Being rigorous about the notation and the terminology is also a way to empower students to recognise when words are misused or concepts misrepresented. "By now, there are popular terms that float around quite a bit – think of entanglement – and sometimes it's important to demystify them," says Kammerlander. "When students start to really appreciate the theory and how beautifully it works, buzzwords lose their appeal." Chu agrees about the confusing role that popular coverage of topics related to QM can play. "Saying that an object can be here and there at the same time – well, that's not really what happens. But some students may have heard this kind of statement and come to class with a certain mental picture," she says. She likes the idea that the QM courses give students the means to become weary of imprecise language and gradually reconcile their initial mental images with the correct interpretations.
Anastasiou doesn't exclude the possibility of revising further the department's QM course offer to acknowledge the changing role of this subject within physics and beyond. "There are topics we can only touch on quickly, and we always need to make a selection – it'd be nice to have more room for relativistic quantum mechanics, say, or for introducing multi-particle systems earlier than in Quantum Mechanics II." As it stands, quantum mechanics is both a lively research area and an ever-stimulating teaching subject.
Quantum mechanics courses at ETH Zurich
Physics III is a second-year BSc course that’s compulsory for all physics students.
Quantum Mechanics I is a third-year BSc course that’s compulsory for all physics students.
Quantum Mechanics II is a third-year BSc core course for the Theoretical Physics curriculum.
These courses are also accessible to mathematics BSc and MSc students; Quantum Mechanics I and II are also accessible to quantum engineering MSc students.
Quantum Field Theory I and Quantum Field Theory II are MSc core courses for the Theoretical Physics curriculum.
Quantum Information Processing I and Quantum Information Processing II are MSc core courses for the Experimental Physics curriculum; both are also accessible to students from various other degree programmes.
Quantum Information Theory is an MSc elective course for the Theoretical Physics curriculum.
Quantum Physics for Non-Physicists is a course designed for the Master in Quantum Engineering that can also be taken by computer science students.